Another intriguing feature of qubits is entanglement. When qubits become entangled, the state of one qubit instantly correlates with the state of another, no matter how far apart they are. It’s as if they’re dancing in perfect sync, sharing information faster than the speed of light—a phenomenon that baffled even Einstein.
To perform calculations, quantum computers use quantum gates, analogous to classical logic gates but operating with quantum principles. These gates manipulate qubits’ superposition and entanglement states, allowing quantum computers to perform massively parallel computations on a scale that classical computers could only dream of.
The potential applications of quantum computers are vast and groundbreaking. They promise to revolutionize fields like cryptography, materials science, drug discovery, and artificial intelligence by solving complex problems exponentially faster than classical computers.
As researchers continue to unlock the mysteries of quantum mechanics and refine quantum computing technologies, the future holds immense promise for these extraordinary machines to reshape the landscape of computing as we know it How Do Quantum Computers Work? .
Unraveling Quantum Computing: The Future of Supercomputing
At its core, quantum computing harnesses the principles of quantum mechanics to process information in ways that classical computers cannot. Unlike classical bits that are binary and can only be in one state (0 or 1) at a time, quantum bits or qubits can exist in multiple states simultaneously through a phenomenon known as superposition. This allows quantum computers to perform vast numbers of calculations simultaneously, exponentially increasing their processing power with each qubit added.
Moreover, quantum computers leverage another quantum principle called entanglement, where qubits become interconnected regardless of the distance between them. This phenomenon enables quantum computers to perform operations much faster than classical computers on certain types of problems, such as cryptography, optimization, and complex simulations.
The potential applications of quantum computing are far-reaching. In healthcare, quantum computers could revolutionize drug discovery by modeling complex molecular interactions in a fraction of the time it takes today. In finance, they could optimize investment portfolios or simulate economic models with unprecedented accuracy. Even in cybersecurity, quantum computing has the potential to crack current encryption methods while also offering new, quantum-safe encryption solutions.
Despite its promise, quantum computing is still in its infancy. Building reliable qubits that maintain their quantum state long enough to perform meaningful calculations remains a significant challenge. Researchers and tech giants like Google, IBM, and Microsoft are racing to overcome these hurdles, investing heavily in quantum hardware and algorithms.
As we unravel the mysteries of quantum computing, its impact on various industries and scientific fields is undeniable. The journey towards practical quantum supremacy—where quantum computers outperform classical ones on real-world problems—is both thrilling and complex, promising a future where computational boundaries are pushed beyond imagination.
Inside Quantum Computers: Harnessing the Power of Quantum Mechanics
Imagine a realm where traditional laws of physics seem to bend, where bits are not just 0s and 1s but can exist in a superposition of states—welcome to the fascinating world of quantum computers. These revolutionary machines operate based on principles of quantum mechanics, promising computing capabilities far beyond those of classical computers.
At the heart of quantum computers lie qubits, the quantum counterparts to classical bits. Unlike classical bits that are either 0 or 1, qubits can exist in a state known as superposition, meaning they can be both 0 and 1 simultaneously. This inherent duality allows quantum computers to process vast amounts of data in parallel, performing complex calculations at speeds unimaginable to classical systems.
Another mind-bending concept essential to quantum computing is entanglement. When qubits become entangled, the state of one qubit instantaneously influences the state of another, regardless of the distance separating them. This phenomenon enables quantum computers to achieve unprecedented levels of connectivity and computational power, paving the way for solving problems that are currently beyond the reach of classical computers.
Harnessing quantum mechanics for computing purposes comes with its challenges. Qubits are delicate and prone to decoherence, where external interference causes them to lose their quantum state. Overcoming decoherence is crucial for the practical realization of quantum computers and remains a focus of intense research and development.
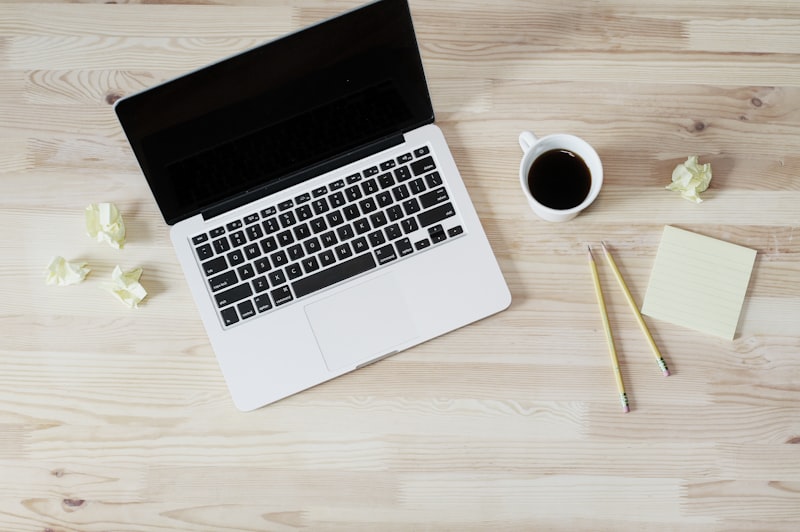
Quantum computers represent a paradigm shift in computing technology, leveraging the counterintuitive properties of quantum mechanics to solve complex problems efficiently. As researchers and engineers continue to push the boundaries of quantum computing, the future holds promise for transformative advancements that could reshape industries and our understanding of computational possibilities.
Decoding Quantum Computing: Breaking Down Complex Algorithms
Imagine a traditional computer as a skilled juggler, adept at handling one ball at a time with precision and speed. Now, picture a quantum computer as a magician who can juggle an infinite number of balls simultaneously, each one representing a possibility or outcome. This analogy captures the essence of quantum computing – harnessing the power of quantum mechanics to perform computations that are simply beyond the capabilities of classical computers.
At its core, quantum computing leverages qubits, the quantum equivalent of classical bits. Unlike bits, which store information as either 0 or 1, qubits can exist in a state of 0, 1, or both simultaneously due to the principle of superposition. This ability to hold multiple states allows quantum computers to explore many solutions at once, making them exponentially faster for certain types of problems.
But that’s not all. Quantum computing also relies on another quantum phenomenon called entanglement. Imagine two qubits becoming entangled, where the state of one qubit instantly influences the state of the other, no matter the distance between them. This interconnectedness allows quantum computers to process information in ways that are not only faster but also deeply interconnected, mimicking the complexities of nature itself.
So, what can quantum computing actually do? Imagine cracking encryption that would take classical computers millennia, optimizing complex logistical operations, or simulating molecular structures for drug discovery with unprecedented accuracy and speed. These are just a few examples of how quantum computing could transform industries ranging from finance to healthcare.
As we decode the potential of quantum computing, it’s clear that we’re on the brink of a technological revolution. The journey from theory to practical application is ongoing, with researchers and companies worldwide racing to harness the full power of quantum mechanics. The implications are profound, promising a future where the impossible becomes routine, and where computational boundaries are pushed beyond anything we can currently fathom.
The Quantum Leap: Exploring the Mysteries of Quantum Computers
Unlike classical computers that use bits of 0s and 1s, quantum computers leverage qubits that can be 0, 1, or both at the same time. This phenomenon, known as superposition, allows quantum computers to process vast amounts of data simultaneously, exponentially increasing their computing power. It’s akin to exploring multiple paths at once and swiftly arriving at the correct solution.
Another mind-bending concept in quantum computing is entanglement. When qubits become entangled, their states are correlated with each other regardless of the distance between them. This means that changing the state of one qubit instantaneously affects the state of another, no matter how far apart they are—a phenomenon Einstein famously described as “spooky action at a distance.”
The potential applications of quantum computers are staggering. They could revolutionize fields like cryptography, drug discovery, material science, and artificial intelligence. Imagine cracking currently unbreakable codes, simulating complex molecules for drug development, or optimizing supply chains with unprecedented efficiency—all achievable with quantum computing.
However, quantum computers are not without challenges. They require extremely controlled environments to maintain the delicate quantum states of qubits. Scientists and engineers are tirelessly working to overcome these hurdles to bring practical quantum computers into reality.
Quantum computing represents a frontier where the rules of classical physics no longer apply. It holds the promise of transforming industries and pushing the boundaries of what’s possible in computing. As research and development continue to progress, we are on the brink of witnessing the full potential of quantum computers unfold before our eyes.
From Qubits to Algorithms: Understanding the Basics of Quantum Computing
Imagine each qubit as a flexible entity capable of exploring numerous possibilities at once, akin to a skilled juggler effortlessly handling multiple balls in the air. This property allows quantum computers to solve complex problems that classical computers struggle with, such as cryptography, optimization, and simulations of quantum systems.
At the heart of quantum computing lies quantum algorithms, specialized instructions designed to harness the unique capabilities of qubits. These algorithms differ significantly from classical ones, utilizing principles like quantum parallelism and entanglement to achieve computational feats that seem almost magical.
One such algorithm is Shor’s algorithm, celebrated for its ability to factorize large numbers exponentially faster than classical methods. This breakthrough could revolutionize cryptography by rendering existing encryption methods obsolete.
Another notable algorithm is Grover’s algorithm, which accelerates the search process for an unsorted database quadratically faster than classical algorithms. It’s akin to finding a marked card in a shuffled deck far quicker than by traditional means.
Understanding these algorithms requires a shift in perspective, akin to viewing a complex puzzle through a multidimensional lens. They represent the synergy between theoretical quantum mechanics and practical computing applications, paving the way for a future where computational boundaries are continually pushed.
Quantum Supremacy: A New Era in Computing Technology
In the realm of computing technology, a groundbreaking advancement is paving the way for a new era: Quantum Supremacy. This concept represents a monumental leap forward from traditional computing methods, promising unprecedented processing power and capabilities. But what exactly does Quantum Supremacy entail?
At its core, Quantum Supremacy harnesses the principles of quantum mechanics to perform computations that would be practically impossible for classical computers. Unlike classical bits that represent information as either 0 or 1, quantum bits or qubits can exist in a state of 0, 1, or both simultaneously due to a phenomenon known as superposition. This unique property allows quantum computers to explore countless solutions simultaneously, making them exponentially faster at solving certain types of complex problems.
Imagine a classical computer as a skilled archer firing arrows one at a time to hit a target, while a quantum computer is akin to an archer releasing a barrage of arrows that can simultaneously explore multiple paths to find the bullseye. This capability is particularly advantageous for tasks such as cryptography, optimization, and simulating quantum physics, where traditional computers struggle with the sheer complexity and computation time required.
Google’s 2019 claim of achieving Quantum Supremacy with its 53-qubit quantum processor marked a significant milestone in this field. It demonstrated that a quantum computer could solve a specific problem in just 200 seconds, a task that would take the world’s most powerful supercomputers thousands of years to complete.
Looking ahead, Quantum Supremacy holds immense promise across various industries, from drug discovery and materials science to financial modeling and artificial intelligence. Researchers and tech giants worldwide are investing heavily in advancing quantum computing technologies to unlock its full potential.
Quantum Computing Demystified: Key Concepts and Applications
Imagine each qubit as a flexible entity, capable of existing in multiple states simultaneously through superposition. This property allows quantum computers to explore numerous possibilities at once, vastly speeding up certain types of calculations. It’s akin to exploring multiple paths in a maze simultaneously rather than one by one.
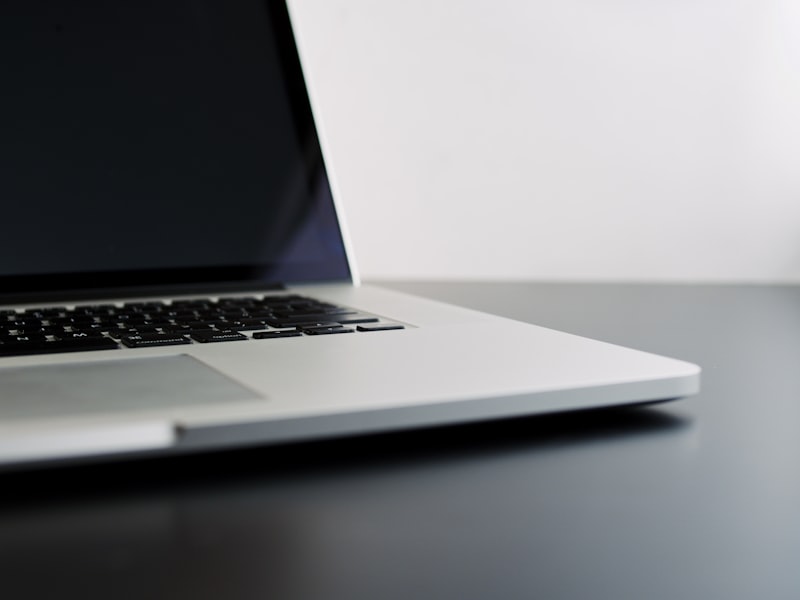
Applications of quantum computing span various domains. In cryptography, for instance, quantum computers could potentially crack existing encryption methods currently considered secure by classical standards. Moreover, in optimization problems, such as finding the most efficient route for deliveries or minimizing energy consumption in complex systems, quantum computers offer unparalleled efficiency.
Looking ahead, industries are investing heavily in quantum computing research to harness its transformative potential fully. From simulating molecular interactions to developing robust AI algorithms, the impact of quantum computing is poised to reshape our technological landscape.
In summary, quantum computing stands at the forefront of innovation, blending theoretical concepts with practical applications. As researchers and engineers delve deeper into its possibilities, the world awaits the dawn of a new era in computational power and problem-solving capability.